“Photonics West_2002”
Holographic Materials VIII,
Monday 21 January
Hilton Hotel:Almaden Room
II……3:30 pm
Report 4659-59
Selfdeveloping dichromated
gelatin thick layers:
manufacturing and control
Alexander Malova,
Nadya Reinhandb, Yury Vigovskyc,
Yulia Zagainovaa, Serge Malovd,
Igor Bogdand, Irina Semenovae
aIrkutsk
State University, Gagarin bul., 20, Irkutsk, 664003, Russia
bInstitute
of Mechanical Engineering Problems of the Russian Academy
of Sciences,
V.O., Bolshoi pr., 61, St.Petersburg,
199178, Russia
cMeDia Ltd.,
Novoslobodskaja str. No 31/1, Moscow, 103055, Russia
dLaser Physics
Institute of the Siberian Branch Russian Academy of Science,
Irkutsk Office,
a/ya 4038, Irkutsk, 664033,
Russia
e A.F.Ioffe
Physical Technical Institute of the Russian Academy of Sciences,
Politechnicheskaya, 26, St.Petersburg,
194021, Russia
e-mail: malov@physdep.isu.ru
The work was supported by Russian
Basic Researches Fond project No 01-02-17141 and European
Office of Aerospace Development (USA) project No 2057P.
2002 is the 150-years anivessary
of the Talbot’s discovery of the dichromated gelatin light
sensitivity.
In 1968, Shankoff proposed
to record hologram using layers of dichromated gelatin (DG)
and demonstrated the possibility to produce highly efficient
DG holograms through the stage of fast dehydration of a recording
layer with propanol. Dichromated gelatin is a practically
ideal phase holographic recording material, which makes it
possible to approach the theoretical limiting value of the
DE and to record more than 1000 superimposed holograms. However,
the low photosensitivity of DG photomaterials as compared
with silver-halide photoemulsions and the low reproducibility
of the results of recording with DG holograms restrict applications
of DG media to predominantly the replicating and printing
of holograms and diffraction optical elements. Record magnitudes
of phase modulation of a relief image, high transmittance,
ultralow noise level, and a high resolution that can be achieved
with DG layers still keep DG in the row of materials that
hold much promise for recording image holograms.
In the middle 1970th
Nakashimura, Inagaki and Nishimura are improved the processing
of hardened DG by adding a treatment with hydrochloric acid
aqueous solution. The HCl processing yelds to the light sensitivity
ten times greater than that obtained by the Shankoff-method.
Namely, a processing of hardened DG is improved by increasing
the dissolution power of a gelatin layer in diluted hydrochloric
acid solution, and, as a result, holograms obtain high light
sensitivity and high reproducibility. Holograms treated with
HCl processing are recorded in the non-linear exposure region
of diffraction efficiency and only on the high hardened gelatin
layer.
In 1970, Erko and I used the
HCl processing for investigation of the recording mechanism
in DG layers. For it the hologram in the unhardened DG was
treatment with HCl-bath and long time washing in the warm
water and vacuum dehidratation of DG layer. The result was
the next- fig. 1.
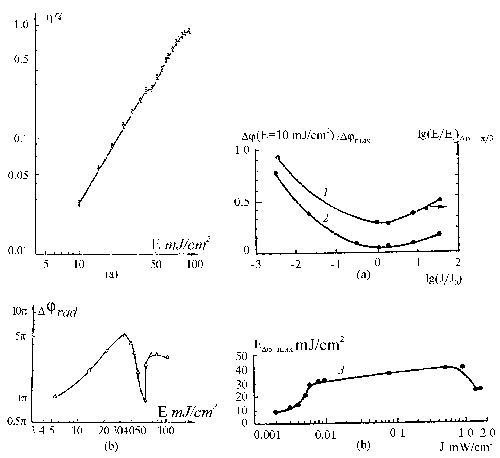
Fig. 1. Hologram recording
in DG layer.
à
– The latent holographic image DE
at spatial frequency equal to 5 lines per mm,
á
– The phase relief of the same hologram
on the 0,63 mkm wavelight.
The recording wavelength was the 0,44
mkm.
|
Fig. 2. The relief parameters
of the recording gratings dependce on the recording
light intensity for 0,44 mkm wavelength.
1 – the exposure nedeed
for the phase relief equal to D
j =p
/3 rad dependence on the recording light intensity
(Å0=mJ/cm2
; I0=0,5 mW/cm2);
2 – the phase relief value
for Å=10
mJ/cm2 divided to maximal
phase relief value in dependence on the recording light
intensity;
3 – the exposure energy for the maximum
phase relief dependence on the recording light intensity.
|
The primary photochemical
reaction is two channel process, and the each channel is connected
with different valency degree of the chromium ions. The relations
between this channel is dependce on the spatial frequency
and the recording light intensity. After developing process
the phase relief has the two maxima form.
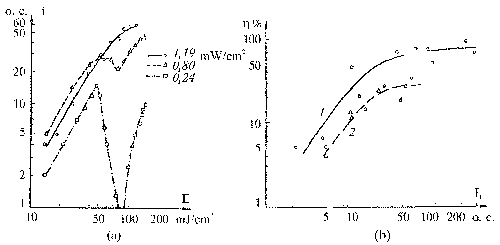
Fig . 3. Hologram recording
in DG layers for 1940 lines per mm.
à – the
latent image DE at spatial frequency equal to 1940 lines
per mm;
á – the
DE dependce on the intensity of the first order of the
diffraction on the latent image for the testing wavelenght
0,63 mkm: 1 – the exposure energy is less than nessesery
for the maximum DE of the latent image; 2 - the exposure
energy is more than nessesery for the maximum DE of
the latent image.
|
For the high DE hologram recording
the best regim is to exposure only up to first maximum for
latent image. The DE of the DG holograms depends on the light
recording intensity, too/
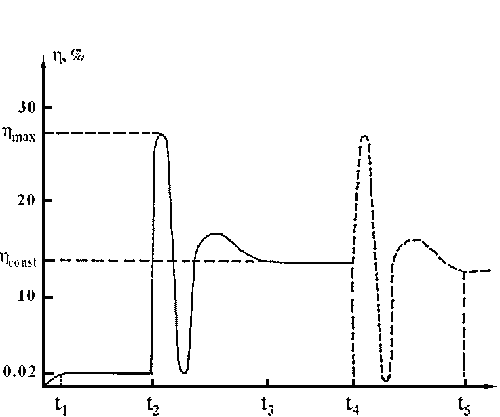
Fig. 4. The hot water vapour
development of the DG layer.
h -
the diffraction efficiency of the holographic gratings
recorded at 0,44 mkm wavelength and spatial frequency
50 lines per mm.
(0-t1 ) – the recording process
up to h =0,02%.
(t1 -t2 ) – the
vapour development process up to h
max.
(t>t4) – the second (postprocessing)
vapour develop.
The recording intensity was near 1mW/cm2.
|
The amplification of a latent
holographic image in DG may be achived by means of the hot
water vapour development. In this case vapour development
leads to modulation of a surface relief (layer thickness is
changed) but not to variation of refractive index inside the
layer. This circumstancees limits the maximal spatial frequency
recorded on the layer by a value of 300 lines per mm. The
vapour developing process has the good reproducibility and
may be used for a real-time testing of DG layers for holography.
The main distinguishing feature
of DG as a polymer is associated with the natural origin of
gelatin, which is produced as a result of the denaturation
of a collagen whose macromolecules have a structure of a triple
hyperhelix (fig. 5). Therefore, the native state of a gelatin
macromolecule also has a biohelix-like structure, which makes
n possible to prepare DG layers with an initially ordered
(helical) molecular structure. In the process of development,
a phase transition from the ordered (quasi-crystalline) state
to a disordered (quasi-amorphous) state occurs under the action
of light and water at all the levels of structure organization
of a gelatin layer, which leads to large photoinduced changes
in optical characteristics of a DG layer.
Fig. 5. Transformation
of collagen into gelatin in the course of denaturation: (a)
triple-helix collagen molecule (hyperhelix), (b) breaking
of noncovalent bond in a collagen molecule, and (c) a polypeptide
helix transforms into separate glomerules (trimers, dimers,
and single gelatin chains).
Fig. 6. Secondary
structures of the crest of a polypeptide macromolecule: (1)
glomerula, (2) a -helix and
its notation, (3) b -fold and
its notation, and (4) reverse point.
In other words, the synthesis
of a DG layer before the recording process is accompanied
by the appearance of stresses applied to "springs" representing
the helical fragments of gelatin macromolecules (fig. 6).
Then, the action of light plays the role of a trigger, which
sets these springs free. The energy stored in these springs
induces a cascade of phase changes in the state of a gelatin
layer. These phase changes give rise to variations in the
macroscopic characteristics of a recording medium
ÒÍE LEVELS OF STRUCTURE ORGANIZATION
OF A GELATIN LAYER
Gelatin is a highly asymmetric
linear polypeptide polymer of protein nature. Macromolecules
of gelatin consist, on the average, of 500-600 amino acid
radicals. The molecular mass of gelatin macromolecules ranges
from 40 000 to 1000 000. Gelatin is a high-molecular compound
characterized by its ability to form various hypomolecular
structures.
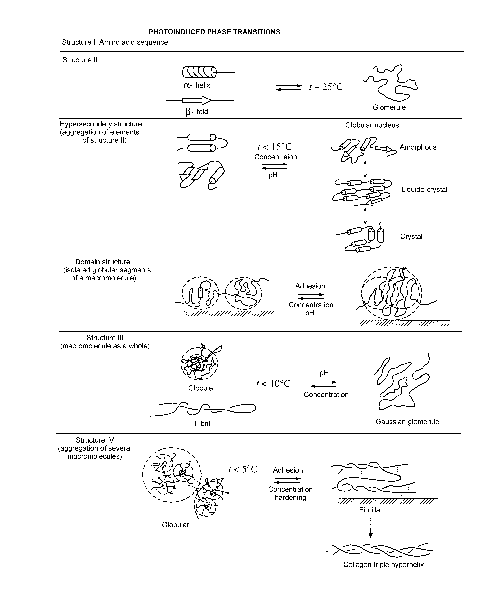
There are six basic levels
in the structure organization of proteins (Fig, 7 ). The
primary structure is determined by the composition and
the sequence of amino acid radicals in a macromolecule of
the polypeptide chain.
The secondary structure
is determined by the configuration and relative spatial arrangement
of protein macromolecules, which have the shape of helixes,
folds, or glomerules (fig. 6).
The hypersecondary structure
is determined by the aggregation of secondary-structure elements
into a single macromolecule, which is manifested, in particular,
as the phase state of the globular nucleus.
The next organization level-
the domain structure is determined by external parameters
(the thickness of a layer, the degree of adhesion of the layer
to a substrate, etc.) responsible for the formation of separate
and relatively weakly bonded globular segments of a macromolecule.
The ternary structure
is determined by the conformation of protein helixes in the
form of fibrils or globules.
The quaternary structure
is determined by aggregation of protein fibrils and globules
and. similar to the domain structure, is highly sensitive
to external conditions (the conformation of a collagen-like
triple hyperhelix is the limiting case of the quaternary structure
for gelatin macromolecules). Distinguishing between the levels
of structure organization of macromolecules, we assume that
interactions of different types have distinct boundaries,
and nonadjacent elements in the molecular chain or elements
of different levels do not interact with each other.
Collagen - a family of natural
polymers of protein nature - is a primary aggregate of gelatin
macromolecules in living organisms, which provides the starting
material for the formation of gelatin. The secondary structure
of collagen has a shape of a left-handed hyperhelix consisting
of three helical gelatin macromolecules. The chemical composition
and physical and mechanical properties of gelatin strongly
depend on the prehistory and the ways of transformation of
collagen into gelatin. The main stages of transformation of
collagen into gelatin involve the breaking of transverse bridge
bonds between polypeptide macromolecules, resulting in the
formation of tropocollagen, and separation of helical chains
in protein (Fig. 5). The molecular mass of gelatin macromolecules
is equal to approximately one-third of the molecular mass
of initial collagen.
Secondary structures of gelatin
Destruction of a triple helix
of collagen is accompanied by the formation of single polypeptide
chain macromolecules. In certain cases, monomer (a
-component), dimer (b -component),
and trimer (g -component)
fractions may also arise (fig. 5). The molecular masses of
a -, b
-, and g -components of gelatin
are related to each other as 1 : 2 : 3. The existence of three
fractions of gelatin with a considerable difference in their
molecular masses indicates the polymolecular nature of gelatin
and the nonuniformity of its properties. Gelatin with a higher
uniformity is characterized by a higher quality. Monomolecular
a -gelatin with a molecular
mass of 40 000-100 000 possesses the best properties. To estimate
the quality of gelatin in the synthesis of DG layers, one
can employ the viscosity coefficient of aqueous solution of
gelatin.
The secondary structure of
the crest of a polypeptide macromolecule is usually considered
as consisting of a glomerule, a
-helix, b -fold, and reverse
points (Fig. 6). On the average, protein molecules contain
35% of a -helixes, 15% of b
-folds, 25% of glomerules, and 25% of reverse points. The
mean length of an of a -helix
is equal to 17 Å, and the sizes of a of b
-fold are usually 20x25 Å. The overall linear size of
a macromolecule may reach 30 000 Å with a diameter of
14 Å. The a -helix-glomerule
phase transition at the level of the secondary structure occurs
within the temperature range of 20-25°C.
In the biochemistry of proteins,
a - and b
-structures are considered as nuclei for the coagulation of
protein macromolecules into a compact (globular) conformational
state. Reverse points play a passive role in the process of
coagulation, forming fragments with minimum resistance to
nonvalent forces that tend to bend the chain of a macromolecule
as a whole. The functional activity of a protein macromolecule
is determined by fragments free of a
- and b -structures. Note that
a -helixes, which are produced
due to interlink hydrogen bonds, are characterized by more
local interactions than b -folds.
Therefore, chromium ions introduced into a gelatin layer at
the stage of preparation of DG films are localized with the
maximum probability near glomerate segments of macromolecules
and near reverse points.
Hypersecondary and domain structures
of gelatin
The formation of secondary
a -and b
-structures occurs immediately after the biosynthesis of a
macromolecule. Next, a -helical
cylinders are packed in an energy-favorable manner. A crystal
is the state with the minimum free energy for a large number
of identical units. The dynamics of coagulation or assembling
(packing) of secondary structures can be interpreted as the
motion of separate segments of a macromolecule. The introduction
of the domain level for the description of the structure of
a macromolecule is dictated by the necessity to take into
account external parameters, including adhesion to a substrate
and limited sizes of a pore where a molecule is located. From
the general physical standpoint, the existence of domains
within a macromolecule implies that a quasi-crystalline structure
can be produced within a separate fragment of a macromolecule.
Ternary structures of a gelatin
layer
If the intermolecular interaction
of two neighboring protein molecules is stronger than the
interaction of separate fragments within a structure unit,
then linear fibrillar structures can be produced. In the case
when the interaction inside a structure is stronger than the
intermolecular interaction, globular forms of protein may
arise (Fig. 7). Both of these ternary hypomolecular structures
have a monomolecular character. Therefore, native (natural)
proteins are divided into fibrillar and globular ones. Conformational
transitions between the ternary hypomolecular structures are
reversible. The condition for such transitions is a viscous-flow
or dissolved state of proteins. Both of the ternary conformations
specified above are characteristic of gelatin. The globular
form is preferable in dilute solutions. In strong solutions
and gels, the considered conformations coexist, but the fibrillar
form is predominant. The fibrillar conformation predominates
in thin gelatin films on substrates ensuring a high adhesion
of gelatin.
Quaternary structures of a
gelatin layer
Formation of coarsely dispersed
ternary gelatin structures results in the appearance of boundaries
between these structures, and the action of surface forces
leads to the aggregation of ternary hypomolecular structures,
i.e., formation of quaternary structures (Fig. 7). The forces
of surface interaction are of the same nature as the forces
of intermolecular interaction. The sizes of protein aggregates
range from 1 to 10 m m, which
allows us to classify these aggregates as colloidal particles.
Ternary and quaternary structures of gelatin determine physical
and mechanical, viscosimetrical, and surface properties of
gelatin films.
CONTROL OF THE STRUCTURE OF
A GELATIN LAYER
Macroscopically, in the preparation
of a DG layer, gelatin as a polymer system passes through
a sequence of different aggregate states. The initial state
in this sequence is a dilute solution of gelatin in water,
where macromolecules reside in the state of a Gaussian glomerule,
or a globule. In the process of DG-layer preparation, as the
solution is poured onto a substrate, the interaction of chain
macromolecules gives rise to the formation of gel, which may
feature properties of a liquid crystal in the case of rigid-chain
molecules or properties of a strong solution with equal volume
portions of a solvent and a polymer.
As the solvent (water) is
evaporated out of the emulsion poured onto a substrate, macromolecules
return to the native state, forming a collagen-like triple
helical structure. The deformation degree of this structure
is determined by conditions of film formation. In the case
of gelling, the forces acting from the side of a substrate
and conditions of drying lead to the unfolding of macromolecules
into linear structures with simultaneous twisting of segments
of these structures into helixes. Depending on the thickness
of emulsion applied to a substrate, films obtained by molding
through gelling on solid substrates feature a planar orientation
of their structure elements. Obviously, the state of structure
elements in a film depends on the state of these elements
in emulsion solution. Specifically, gelatin in a film obtained
from solution at a temperature higher than 35 °C has a conformation
of a Gaussian glomerule and does not display any features
of ordering and planar orientation of structure elements.
The degree of adhesion of emulsion to a substrate can be controlled
with the use of a gelatin sublayer with a thickness of 0.5-1
mkm having a variable degree of hardening or a sublayer of
a 5-10% solution of sodium or potassium silicate (liquid glass).
Thus, in synthesizing and
pouring DG layers, one can control the structure of a gelatin
layer. Below, we describe procedures of such a control.
DG layers with the maximum
helicity of the secondary structure
Layers of dichromated gelatin
developed with the use of a standard technique or by water
vapor were prepared in the following manner.
An aqueous solution of gelatin
with a weight content of dry gelatin from 0.5 to 6% was filtered,
degasified, and poured simultaneously with cooling down to
30-35 °C onto a glass substrate heated to the same temperature.
The substrate was placed horizontally on a heated thick glass
sample. For gelling, the plate poured with solution was kept
in a cooler at a temperature of 5-10°C during 4-6 h. The thickness
of the DG layer thus prepared mainly depends on the initial
concentration of gelatin solution. The DG layer was sensitized
in an aqueous solution of ammonium bichromate at a temperature
no higher than 20°C during 5-7 min. The concentration of ammonium
bichromate should not exceed the initial concentration of
gelatin solution.
Otherwise, crystal structure
may appear in a DG layer, and the intrinsic light scattering
coefficient may increase for such a layer.
Preparation of DG layers with
the temperature regime specified above ensures gelling with
the maximum helicity degree of the secondary structure state.
The minimum layer thickness, which can be achieved with low
gelatin concentrations, allows one to prepare a gelatin layer
with the maximum fibrillarity degree of the ternary structure.
The required level of pre-exposure hardening of a gelatin
layer is achieved with an appropriate dose of illumination
of the prepared and sensitized DG layer with a UV lamp or
sunlight.
DG layers with a globular structure
For the preparation of thin
gelatin layers with a globular structure, 0.2-1.0% aqueous
solution of gelatin was poured onto a substrate cooled down
to 5°C and then evaporated in a vacuum in accordance with
the technique described above. For higher (up to 5%) gelatin
concentrations, up to 20-30% of isopropyl alcohol was added
to gelatin solution to prevent gelling (the Henderson method]).
Then, such a solution was poured onto a substrate and dried
in a vacuum.
For preliminary hardening,
a 5% alcohol solution of hinone was added to gelatin solution
before evaporation, which made it possible to produce a globular
layer of nongelling gelatin.
To ensure a high intrinsic
photosensitivity with respect to red light, ammonium bichromate
(5-100% of the weight of dry gelatin) was introduced into
gelatin solution. Upon the addition of ammonium bichromate
to gelatin solution, all the procedures were performed under
illumination with weak red light.
The holograms registered in
photosensitive layers of several millimeters thickness have
a number of specific properties, the most important of which
are extremely high spectral and spatial selectivities. These
highly selective gratings are used for the development of
modern optical information processing devices, in optical
memory systems with multiplex information recording and for
interfiber connectors in optical telecommunication networks,
for the variety of spectral filters for imaging, etc.Usual
holographic materials can not be applied for recording of
grating of millimeter thickness, because it is impossible
to maintain the material uniformity during the post-exposure
processing, Differential shrinkage caused by post-processing
leads to the reduction of diffraction efficiency, and besides
to the asymmetry and widening of selectivity contour of output
signal. Selfdeveloping dichromated gelatin is one of the solutions
to overcome these problems because it does not require post-exposure
processing.
In the middle of the 1980th
we realized new type of DG-system - selfdeveloping dichromated
gelatin or gelatin-glycerol emulsion, which is not solid state
media unlike ordinary DG. The developed photosensitive medium
differs from the wellknown dichromated gelatin by the addition
of certain amount of glycerol. Glycerol in these layers serves
as the developing component and also as the plastic component.
It keeps some amount of water molecules that are carrying
out the developing process due to their hydrogen ties. And
besides glycerol provides enough plasticity to the material
to make layers of millimeter thickness.
However several problems arise
at synthesis of superthick (more than 0,5 mm) layers. We have
to find the reasonable limit of the emulsion drying time.
Very long drying (more than three day) time is preferable,
it leads to better gelatination and "ripening" of colloidal
medium. However, long time drying is not suitable from the
technological point of view. Our attempts to solve this by
conventional methods such as ventilation by hot air or alcohol
dehydration were not successful, they result in originating
of a strong gradient of optical refraction in the layer depth.
The processing of colloidal layers by microwave radiation
resulted in their flaking from the substrate and to unmonitored
change of the conformation gelatin macromolecules status.
Synthesis of selfdeveloping
dichromated gelatin layers.
The procedure of the sample
preparation is the following:
the gelatin is solved during
1hour in water (1 g of gelatin on 5 ml of distilled water)
at temperature 50oC, then glycerol (0.8 ml in 5
ml of water) was added, and the solution was maintained at
temperature 40oC within 2 hours.
Then we added ammonium bichromat
of necessary concentration (20 % of the weight of dry gelatin
or 0.2 g in 5 ml of a water) in the obtained homogeneous solution.
Then ammonia for achievement of pH=9.0 was added, then the
stain methylene blue (MB) as solution of 10 mg of the stain
in 100 ml of water was added.
The prepared emulsion solution
filled up the transparent pan with lateral walls of the necessary
altitude (from 0.5 up to 3mm), and it was covered by special
glass. Gelatination procedure was carried out at the temperature
of 25oC within 24 hours. In outcome the layer of
the given depth was received.
KCl doped emulsions were tested
to investigate the possibility of chemical control of the
SD DG structure. KCl was added during the emulsion preparation
in the amount of several percent.
We used the gelatin sublayers
to investigate the influence of adhesion from substrate. The
substrate was covered with 1% gelatin water solution. After
drying the sublayer of about 1 micron thickness was obtained.
Then it was hardened in the Orwo-400 solution during the various
time intervals to achived the sublayer different hardness.
It is important to take into account the influence of substrate
properties in variety of applications, particularly, for interfiber
connectors, where the substrate role is played by bridged
fibers.
The water structure and it’s
control by means of the IR laser annealing
The SD DG system contains
water in quantity sufficient for the hologram development.
The water in such system is held with the help of glycerol
and consequently the holographic properties depend on glycerol
concentration in a system. This system contains great many
of water, and, therefore it is possible to consider it almost
as aqueous solution, to which the quasicrystal liquid structure
role is essential. The collective character of the water molecule
motion is confirmed by the molecular dynamics results.
It is possible to influence
on SD DG structure in all volume by means of the laser IR
radiation. IR electromagnetic radiation causes molecular rearrangement
in the material. This effect consists in the loss of coordinate
oscillations stability: the part of molecules does not return
in the position with initial coordinates. It is impossible
to estimate the optimal radiation wavelength for such annealing
because of composite emulsion structure. Therefore, basing
on the known data on absorption spectrum of water we chose
1 micron wavelength radiation. Besides it has another advantage
that the IR near range radiation passes through the glass
substrate and consequently in thicker layer there are no undesirable
effects of an interference of IR radiation.
Technique of the laser annealing
After holding the emulsion
layer during a day the quasicrystal selfdeveloping dichromated
gelatin structure was formed. Then the plate was subjected
to IR laser radiation with 1 micron wavelength and with known
values of power in the pulse. In order to eliminate the influence
of all transition processes, the hologram was recorded 10-12
hours later.
The holographic characteristics
measurement.
We illuminated samples with
interference pattern constructed by two plane light waves
from the He-Ne laser. The spatial frequency of the recorded
diffraction grating was hundreds lines/mm. The energy of two
beams was 6mW. Diffraction efficiency was measured as the
relation of intensity values of diffracted and input beams.
The IR laser annealing
results
The variable parameters for
the annealing IR pulses are the pulse energy, duration, and
shape. It should be mentioned that maximal diffraction efficiency
obtained in SD DG layers is about 70%. However when we analyzed
the effect of different factors on the diffraction efficiency
we were working in the range of 5-10% efficiency in order
to eliminate the influence of non-linear properties of medium.
The experimental results for
IR laser annealing are shown in Fig. 8 (fig. 1 from the paper)
for holographic grating of 600 lines per mm recorded in selfdeveloping
dichromated gelatin layer of 1mm thickness by radiation of
He-Ne laser. We analyzed the dependence of diffraction efficiency
on the amount of IR laser annealing pulses and full pulses
energy (Ean) and the best result occurred for six
annealing pulses. Figure 8 shows the obtained diffraction
efficiency of holograms increasing – m=[(DEan –
DE0 )/DE0 ]100%, where DEan
is the diffraction efficiency of the IR laser annealing SD
DG test hologram and DE0 is the same for SD DG
test holograms without laser annealing. One can see from this
figure that there is an area of considerable diffraction efficiency
increasing. The best result received at an annealing by six
pulses with duration 3 msec at full impulses energy equal
to 18-30 J. However, the exact energy level depends on the
material thickness, on the concentration of gelatin in emulsion,
and on the type and series of gelatin. It should be pointed
out that all obtained values of diffraction efficiency for
the annealed materials are higher (on average 2-3 times) than
they are for the plates not subjected to the annealing. It
is visible that the diffraction efficiency curve (Figure 1)
has the brightly expressed peak (for annealing energy more
than 20 J) at small pulse duration. Further increase of pulse
energy leads to considerable diffraction efficiency increasing.
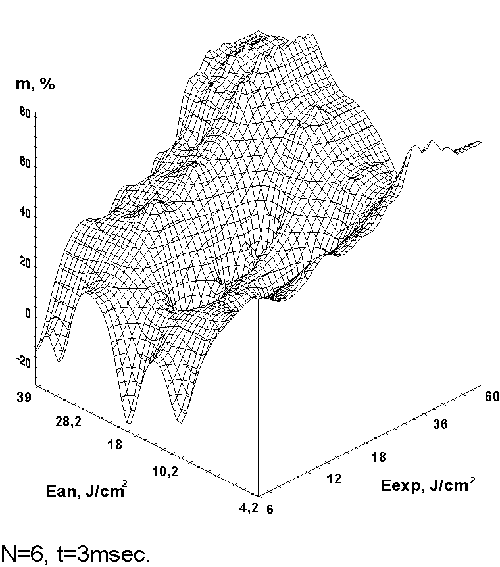
Figure. 8. Diffraction
efficiency of holograms recorded in selfdeveloping dichromated
gelatin layers with different energy of IR laser annealing
pulses with 3 msec duration of six annealing pulses.
The pulse irradiation regime
for laser annealing is preferable, because it allows to estimate
precise energy of effect on the layer structure. The pulse
irradiation is to some extent like sharp system “shaking”,
and the necessary macromolecules packing is reached by the
following relaxation processes in the system.
In our opinion IR electromagnetic
field causes impulsive disturbance of water at the level of
collective modules while single water molecules and gelatin
squirrels do not absorb radiation of 1 micron wavelength9.
Water quasicrystal net rearrangement conducts the change of
electromagnetic field affecting gelatin macromolecules. These
macromolecules move under of this field action and change
therefore tertiary and quaternary conformation status to achieve
an energy minimum in new quasicrystal net. Thus spiral segments
do not change, and this means that the SD DG system photosensitive
properties do not change too. (Our conception consists in
the material photosensitivity based on untwisting of spiral
segments.)
In general our experiments
showed that the variety of pulse parameters affect on the
resulting diffraction efficiency value. The main parameter
is, of course, the amount of energy absorbed by the material.
However, the pulse duration and its shape (this means the
sharpness of fronts) as well as the moment of the annealing
relatively the emulsion maturing time are also of importance.
It is obvious from the experiments that there exist several
solutions to reach the maximal diffraction efficiency by combining
the optimal pulse energy, shape, duration and amount of pulses.
However that concerns the moment of annealing, multiple experiments
showed that the optimum is around 24 hours after emulsion
pouring on substrate
The influence of adhesion
to substrate on the selfdeveloping dichromated gelatin structure
Experiments showed that SD
DG layer separation from glass substrate often occurs causing
the diffraction efficiency reduction. This effect is called
photoinduced collapse of the emulsion at recording. For elimination
of this phenomenon we proposed to utilize an additional gelatin
sublayer with the hardness higher than it is for the photosensitive
emulsion layer. We investigated what optimal value of the
sublayer hardness. The dependence of holographic properties
of the SD DG layer on the substrate hardness is shown in Fig.
9 (fig. 2 from the paper). One can see from the Figure 9 that
the maximal diffraction efficiency was observed for the sublayer
subjected to hardening in ORWO-400 during 4.5 munites (corresponds
to H=4.5).
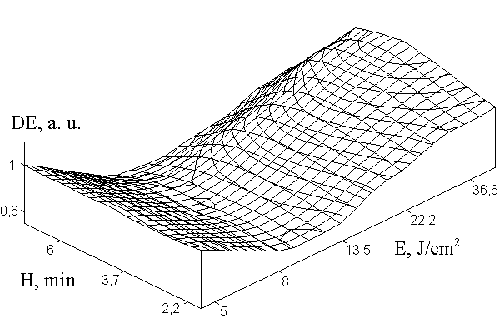
Fig. 9.
Influence of hardening degree Í of sublayer on the SD DG exposition
characteristics.
SD DG emulsions containing
high-molecular-weight polymer
We developed the new version
of selfdeveloping dichromated gelatin, in which 50 % of glycerol
in emulsion was replaced by natural polymer (polysaccharides
similar to stratch) obtained from plants, its molecular mass
is about 20,000. The first experiments showed considerable
reduction of the layer gelatination time (Figure10 – fig.
3 from the paper). The formation of quasi-crystal structure
occurs faster in this case compared with initial version of
SD DG. Obtained holograms have improved storage time.
Fig. 10. Influence
of the gelatination time on the SD DG exposition characteristics
for 50% polymer replacement of glycerol.
The SD DG emulsion doped by
KCl
We investigated the influence
of salt addition to the composition. We used KCl, because
it is known that K ions affect the aquacomplex structure.
The experimental results are shown in Figures 12(a) and (b)
(fig. 4 from the paper). It is found that KCl essentially
improves photosensitivity of the emulsion. The shape of the
diffraction efficiency contours changes under the influence
of KCl, however the character of this change depends on gelatination
temperature schedule.
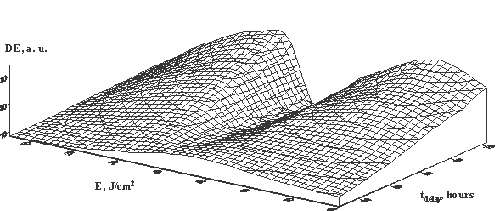
Fig. 11. The
hologram diffraction efficiency for SD DG doped by KCl in
the gelatination time dependence at temperature 24î
Ñ (a) and at temperature 00
Ñ (b).
CONCLUSIONS
We proposed different technologies
to control the holographic characteristics (diffraction efficiency,
storage time) of selfdeveloping dichromated gelatin material.
It was shown that IR laser
annealing before hologram exposure results in increase of
diffraction efficiency.
In order to overcome the problem
of the photosensitive layer separation from the substrate
we proposed to use strongly hardened gelatin sublayers. It
was shown experimentally the improvement of diffraction efficiency
in this case.
It was shown that the storage
time of hologram can be improved by replacement of part of
glycerol by natural polymer with high molecular weight.
Addition of salt to the emulsion
leads to the increase of the material photosensitivity and
to the diffraction efficiency improvement due the influence
of ions on water molecules inside the material.
REFERENCES
- Erko A.I., Malov A.N. Development parameters
optimization of the dichromated gelatin layers for optical
information recording. / Scientific and Applied Photography.
– 1980.- vol. 25 – N 3 – pp. 185-187.
- N.F.Balan, A.I.Erko, V.V.Kalinkin, A.N.
Malov. et al, USSR Patent no. 1347757. 1985.
- V.P. Sherstyuk, A.N. Malov et al.,
“Some principles for formation of self-developing dichromate
media”, Proc. SP1E, 1238, pp. 218-223, 1989.
- Maloletov S. M., Kalinkin V. V.., Malov
A. N., Sherstyuk V. P. On the feasibility of designing “self-developing”
media with high diffraction efficiency. / Scientific and
Applied Photography. – 1991.- vol. 33 – N 3 – pp.
448-455.
- S.P. Konop, A.G. Konstantinova,A.N. Malov,
“Mechanism of the hologram recording in self-developed dichromated
gelatin layers”, Photonics & Optoelectron., 3,
21-29, 1995.
- Consnantinova A.G., Malov A.N., Conop S.P.
The selfdeveloped dichromated gelatin films for holography.
/ Proc. SPIE. – 1996. - Vol. 2969. - pp. 274-277.
- N.Reinhand, Yu.Korzinin, I.Semenova. “Very
thick holograms: manufacturing and applications”, Journal
of Imaging Science & Technology, 41,
3, 241, 1997.
- Vigovsky Yu.N., Malov A.N., Malov S.N.,
Konop S.P. Photoinduced Phase Transitions in Hologram Recording
in Layers of Dichromated Gelatin. / Laser Physics. – 1998.-
vol.8 - ¹ 4,
pp. 901-915.
- Vigovsky Yu.N., Malov A.N., Malov S.N.,
Fetshenko V.S., Konop S.P. New dichromated gelatin technologies
for the diffraction optical elements fabrication. / Proc.
SPIE. - 1998.- vol. 3347- pp.314-324.
- N.Reinhand, Yu.N.Denisyuk, N.N.Ganzherli,
I.Maurer, I Pisarevskaya, V.Markov, “Application of selfdeveloping
dichromated gelatin for holographic data storage”, Proceedings
SPIE(Photonics West’98), 3294, pp.22-30, 1998.
- .Yu.N.Vigovsky, S.P. Konop, A.N. Malov,
S.N. Malov, “Photoinduced phase transitions in layers of
dichromated gelatin“, Laser Physics,. 8, N
4, pp. 901 - 915. 1998.
- L.E. Kruchinin, I.V. Bogdan, Yu.S. Zagaynova,
Yu.N. Vigovsky, A.N. Malov. “Selfdeveloped colloidal recording
media for fiber interconnects and holographic elements fabrications,”
“Proceedings of the First Asia-Pacific Conference on
Fundamental Problems of Opto- and Microelectronics – APCOM’2000”,
SPIE – RFFR: Vladivostok, 2000. pp. 55 – 59.
- L.E. Kruchinin, I.V. Bogdan, Yu.S. Zagaynova,
Yu. N. Vigovsky, A.N. Malov, “Optoelectronic applications
of the selfdeveloped colloidal holographic recording media”,
Proc. SPIE, 4513, pp. 142 – 146, 2001.