The
water-blocking buffer gel structure visualization for the
real fiber cable and the influence distributed defects to
signal loss
Igor V. Bogdan, , Basil V. Levit, Dmitry B. Lipov, Alexander N. Malov, Sergey N. Malov,
Pavel I. Sinica, Nataly Yu. Vigovskaya, Yury N. Vigovsky, Yullia S. Zagaynova
Irkutsk State University,
TCMS-12 Rostelekom Co. (Irkutsk, Russia),
MeDia Ltd. Co., (Moscow, Russia),
Irkutsk office of the Laser Physics Institute of Siberian Division of the Russian Academy of Sciences
(Irkutsk, Russia).
The direct-shadow visualization with the help of a coherent radiation translations the optical fiber module has allowed to detect local optical heterogeneity as in a density of the water-blocking buffer gel and violation of a twist-structure of optical fibers. The optical module parts having bulk distributed transmission imperfections was revealed with the help of standard optical time-domain reflectometer (OTRD). The really detected imperfections identification was carried out, the visual imperfections separation to exploitation and manufacturing defects.
1. Introduction
The spatial distributed imperfections visualization method is additional one to nondestructive techniques for diagnostics and testing of fiber optical line of communication (FOLC), which is allowing to determine the reason of appearance defects. Whether these module imperfections are exploitation or manufacturing. The shadow method of detection of module imperfections allows in a new fashion to cast a glance at the requirements for manufacture of fiber systems on the other aspect. The simplicity and obviousness of the shadow coherent-optical monitoring schemes enables widely to use them for nondestructive monitoring of quality of an industrial production. This method also allows carry out trials in the real season temperature and to prevent emerging a number of imperfections, beforehand showing: under what climatic zones the tested optical modules can be used.
2. Visualization of phase objects
The refractive index of inhomogeneous phase object is function
of three space coordinates
(fig.
1). Under the Snell's law the laser light ray passes a point
on the observed plane, while nondeflected beam would come in a point
.
The laser light ray passed, coming in a point
,
deviates from initial trajectory. Also the light ray passes an optical path
in a heterogeneity, defined from integral
,
where s - geometric path length of a ray in object.
Thus except visual direct observation in the image plane it is possible to measure the following quantities: ray displacement, deflection ones in a heterogeneity, phase shifts. The detection of phase objects by the direct-shadow method is fulfilled without the optical elements because visualization happens due to focusing properties of the
object (the fiber module with water-blocking buffer gel). The parts optical module is illuminated divergent beam from helium-neon laser (fig. 2.).
The light beams, which are taking place through heterogeneity in the water-blocking buffer gel, change the ones direction. It reduces to light intensity redistribution in the image plane (fig. 3.). The formed direct-shadow image depends of absolute value of angular deflection. The alteration of light intensity is proportional to second-order derivative of refractive index
,
where
-
incident light intensity,
-
alteration of light intensity,
-
refractive index.
Quantitative responses can be received with the help direct-shadow method in the event that neglect a ray curvature in a heterogeneity and also additional displacement on a path between a heterogeneity and observed plane, These assumptions are not always admitted for examination in the field of sharp alteration of refractive index. Therefore, this method seldom applied to quantitative measurements. Usually it use for a qualitative detection of zones with great index density of a heterogeneity. The quality of an image can be rose by use a cylindrical lens in the final cascade of image formation. The lens focus length must be in conformity with a diameter of the research module and parameters of a laser beam.
3. The raised attenuation causes
In the winter of 1999-2000 the attenuation increasing was detected at an evaluation of quality of the "Novosibirsk-Khabarovsk" FOLC on some parts in connective couplings AFL, which connected the dielectric Fujikura corporation cable with OPGW cable, which was built in the Alcoa Fujikura corporation storm-rope. The measurements by the OTDR have shown [2], that the attenuation was raised in optical fibers of a dielectric cable, which are placed above a surface of ground and was most subjected to influence of low temperatures (fig. 4.). It is necessary to mark, the imperfection was not precisely location. It was a spatially distributed defects for the space of ten meters. For the first time, the raised attenuation was discovered in January-1998. In January-2000 similar problems was revealed in a dielectric cable on six parts in outcome of test-prophylactic of measurements. A maximum value of attention has made about 5,4 dB. There are some peculiarities such as: the given effect is exhibited at negative temperatures and on a vertically cable part which is going out to support from a high-voltage line.
The most probable reason of the increased signal attenuation in a dielectric cable is represents by local structure heterogeneity origin in the hydrophobic gel compound of the optical module because of thermal blow-up of a gel polymer structure at low temperatures. The gel structure was changed thanks to modification of moisture-matter in the gel layer. But a real changes of gel structure is while unknown in process of exploitation.
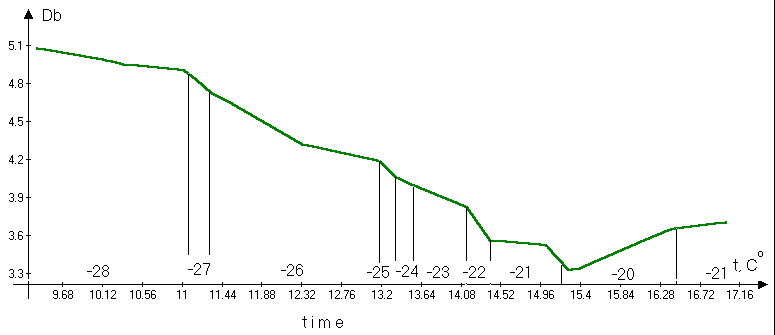
The given situation is complicated by presence
of capillary and boundary effects on interaction of fibers
with gel, located in the module in the form of a thin layer,
and changing its physical properties. The carried out analysis
of the published information [10,11,12] and possible mechanisms
of interaction of "the polymeric compound - solid body" systems
has allowed to allocate four basic working hypothesis variants
about the influence of the surface on the boundary polymeric
layer.
The chemical hypothesis [3] is based on an estimate of interaction of macromolecules with a solid surface, as a result of which chemical and physical bonds may arise . An adsorption of macromolecules on the surface precedes the formation of these bonds at which it is necessary to take into account a competitive adsorption of builders of polymeric compositions on fissile centers of the surface. The course of these processes is bound by an activation of interaction energy of "the polymer compound - solid surface" systems and limited in time on losses of mobility of polymer compound segments at its solidification (or volatilization of a solvent) what can involve the change of compositions and properties of the boundary polymeric layer.
According to the physicochemical hypothesis [4], the change of structure of the boundary layers can happen also owing to effects limiting mobility of molecules or their segments because of orienting influence of a solid surface and other factors. All this causes to a limitation of macromolecules conformations, and from here - the change of structure and properties of the boundary layer in comparison with the polymeric layers removed from the surface.
The deformation hypothesis [5-7] is based on an estimate of the deformed, "stretched" state of boundary polymeric layer. This is conditioned by that the shaping of a layer and origination of adhesion bonds happens at that moment when the polymeric compound is in a rather relative frame state and its macromolecules are liable to accept various conformations. The boundary layer is unable to overcome volume collapse strains and it appears stretched at solidification of a polymeric compound and attendant to this collapse process.
The principle of the thermos - physical hypothesis [4] is reduced to the following. As the process of the polymerization has the exothermic nature in usual, considerable temperature gradient can arise because of a low thermal conduction of the polymeric compound in volumetric samples while this effect is rather insignificant in thin polymeric layers and process of a polymerization is close to isothermal. Therefore a structure of the polymeric compound in the block and in boundary layers may be essentially distinguished, and the properties of the boundary polymeric layer may be different from the properties of the volumetric model consequently.
Apparently, the changes of structure and properties of the boundary layers can happen just as because of cumulative influence of all listed mechanisms of interaction of the polymeric compound with a substrate, so as a result of dominant influence of one of them [8,9].
In the theory of heterogeneous systems it is necessary to differentiate essentially homogeneous phases and surface layers, having assigned the latest terminating thickness [4]. As the influence of each of phases, strictly speaking, is spread to all thickness of the next phase, we have to do, obviously, with effective thickness, outside which the diversion of local properties from their volumetric values becomes incidental. The introduction of this concept becomes possible owing to a smallness of activity radius of intermolecular forces, what causes rather prompt to influence reduction of one of phases on any the next phase property.
It follows from equilibrium conditions of system involving the surface layer and volume that there must be a force in a surface layer which counterbalances forces of an intermolecular interaction in the field of boundary of separating medium [3,4].
This force is designated a surface tension: tensioning a surface layer, this makes it more strong and raises as if its elastic modulus. Consequently, it is possible to judge from a difference of modules quantity of the surface tension.
Thus, the elastic modulus of the surface layer differs from volumetric as a result of a surface tension. From the above reasoning it is possible to estimate pressure of a surface layer of a polymeric compound (gel) considering this interaction as a capillary phenomena, in view of a smallness of radius of a fiber. The additional pressure on the fiber on the source side of a gel can be determined from the law of Laplace at medial curvature of a surface
,
defined by the main radiuses of curvature
and
.
The additional pressure is equal
Where
- surface tension of a boundary layer.
In case of a cylindrical surface
and
,
.
Reasons for a modification of a structure has arose from specific
thermal evolution of boundary layers of a hydrophobic compound in the optical
module in a warmer season. The thermal inter seasons fluctuations are in a limit
from
to
,
and daily variations of temperature about to
.
The non-agreement of thermal, adhesion
and capillary properties is the basic reason for origin of imperfections. Also
the losses in the FOLC can be conditioned by imperfections of fibers, which
are formed by micro bends at buffer tube sizes reduction of a on considerable
part of module at low temperature. The micro bends of fibers are caused by local
variations of a hydrophobic gel compound density, filling the buffer tube. A
losses are increased in micro bends. The surplus geometrical fiber length in
the module and inappropriate thermal expansion of the optical module are conditions
for shaping the increased micro bends number. The extension of the module happens
in a warm season. In the winter the inverse process (compression) is difficult
because of the increased viscosity of a hydrophobic compound. Thanks to it fibers
in buffer tube are twisted in a spiral spontaneously, i.e. take place a twist-structure
of optical fibers.
4. The optical module spatially distributed imperfections detection
The researches of the gel composition properties were carried out. The structural-capillary properties were also taken into consideration such as temperature blow-up of gel, variation of moisture-matter and presence availability of capillary-adhesive occurrences in the gel layer [10]. The optical configuration of visualization and documenting of heterogeneity in the module were specially developed with the of extreme coherent laser radiation for visible light range (fig. 5).
The module was fixed in the scheme (configuration) (fig. 5).
The focusing laser beam with a high intensity (
)
is driving into fiber end-face. This beam was spread in volume of the optical
module instead of on a single fiber. Diffusion a laser radiation on heterogeneity
are "gas bubbles" and weighed inclusions in the gel layer. It is necessary to
mark, the presence of gas bubbles through half-transparent buffer tube (in the
module with half-transparent buffer tube) can be observed visually without use
of the laser.
Gas bubbles by a size of the order
were observed on module part with the length of the order
.
The diffusion only on heterogeneity of the order
was observed at
. Gas bubbles
were observed through an interval
.
The detection of local heterogeneity makes possible the supposition about spatially
distributed imperfections. These local inclusions can be a consequence of
a) violation of the requirements at manufacture of optical cables (introduction of an air and weighed inclusions in the gel);
b) structural modifications of gel at exploitation of the optical cable in the climatic conditions of East Siberia and vertical disposition of an optical cable.
Probably, gas bubbles lead to spatially distributed. These
imperfections can lead to great losses in the FOLC. At a vertical disposition
of a cable gas bubbles by gravity and at high temperature (up to
)
can move in optical module because of diffuse capillary currents of gel inside
the module (in the module). Gas bubbles promote forming of micro bends in fibers.
The existence of gas bubbles and weighed inclusions can lead to gradual attenuation
of a signal for the space of all vertical cable parts.
5. The direct-shadow method
The direct-shadow method was used study of inside structure
of optical module. The optical module fixed vertically in the holder was placed
in a lens focus
(fig. 2).
The holder allows to move the module along a vertical axes. The enlarged image
of the module on a screen was exceeded with the help of objective. As the researched
module has a half-transparent buffer tube, it is possible visually inside the
module.
The constantly varying image as spatially distributed imperfections
of a various type was observed at transition of the module in the holder. It
is necessary to mark, that the imperfections are exhibited the best of all at
dynamic loading. The obtained images were registered. In some cases small-sized
gas bubbles (
) was well observed
(fig. 6(a)). These imperfections can leads to forming of fiber micro bends due
to fiber modification by the gel pressure. Also, bubbles with the sizes by
are observed. These imperfections give a diffusion image of module with clearly
looked fibers systems (fig. 6 (a, b)). Fibers was stuck by gel in these parts
of module with gas bubbles. Fibers in the module form multi fibers communication
system. This configuration of fibers places on a center of the module, that
lead to strain of fibers along their own axes.
Moreover the gas bubbles, the local heterogeneity as of "dark areas" were observed at motion of the module in the holder (fig. 3.2.2.(c, e)). These heterogeneity had more precise boundaries than gas bubbles. "Dark areas" move in module at exerted pressure to one from end-faces of the module. The light scattering is observed on the boundaries of local heterogeneity. Probable the light scattering is scattering volume containing gas molecules. The following reasons of emerging of areas with the increased density were considered.
1. The local heterogeneity can be a consequence of structural modifications of hydrophobic gel compound under an operation of season or daily modifications of temperature.
2. The weighed particles are in hydrophobic gel compound. The weighed particles can be to play role of the crystallization nucleus for gel layer growth layers or for water condensation with the same structural gel modifications.
The weighed transparent particles (presumably - glass crumbs
) were detected at a research of gel with helps of microscope. Size of particles
makes
micron. The assigning
glass crumbs in gel layer remains vague. Probable, areas of weighed particles
destroy fibers due to the friction, which is a consequence of diffuse micro
capillary currents in gel layer inside the module by gravity at vertical disposition.
Also, the inside walls of buffer tube can be destroy that lead to diffusion
of water steam through buffer tube increased of a moisture amount in a gel layer
and structural modifications.
Also, the singularities of a fiber-system disposition in the module were detected with the help of the laser direct-shadow method. On same parts, the joint and twisted fibers into module were clear visible .
Conclusion
As a result of experimental research the following outcomes are obtained:
1. The features in a complete set of optical fibers inside an optical module are detected.
.2. Are found of a different types of actuation in a hydrophobic compound. To the detected defects concern of such local non-uniformity as: gas "bladders" and " dark areas ".
3. The module was checked up not involved in activity on a vertical segment. All above-stated defects were detected. That enables to approve, that at a vertical run of the researched optical module there will be same problems losses on FOLC.
4. In the given article the qualitative description of defects is resulted only. But, it is necessary to mark, that the theoretical description of the detected defects is extremely inconvenient.
The work was supported by Russian Basic Researches Fond project No 01-02-17141 and European Office of Aerospace Development (USA) project No 2057P.
References
- Moskalev V.A. Theoretical principles of the optical-physics researches. – Leningrad, Mashinostroenie, 1987, p .246-253. (in Russian).
- Agrafonov Yu.V., Lipov D.B., Malov A.N., Ovchincin A.V.// Computer Optics, 1999, volume 19, p. 159 – 164.
- Structure and properties of the boundary layers in polymer-solid systems. / Ed. by Yu. S. Lipatov. – Kiev: Naukova Dumka, 1972. (in Russian).
- Rusanov A. I. Phases equilibrium and surface phenomena. – Leningrad: Himiya, 1967. (in Russian).
- Marcuse D. Principles of optical fiber measurements. – London: Academic Press, 1981.
- Sterling D.J. Technician’s guide to fiber optics. – Delmar Publishers Inc., N-Y, 1993.
- Ubidulaev R. The Optical -Fiber Systems. - M., Nauka, 1998.-260c. ( in Russian).
- Kulchin Yu.N. The distributed fiber-optical sensors and measuring nets. 1999, Vladivostok, Dal'nauka ( in Russian).
- Goldfarb I.S. Transfer characteristic of optical cables at action of mechanical loads. // "Electrosvyaz" № 12, 1980. (in Russian).
- Muradjan A.G., Goldfarb I.S., Inosemzev V.P. Optical cables of multichannel communication circuits, "Radio i svyaz", M, 1987 (in Russian).
- Gowar J. Optical communication systems. – London: Prentice/Hall International, 1984.